Literature Review: e-methane (e-LNG) for Shipping
The information included in the literature review to follow includes a mix of peer-reviewed and grey literature sources. Importantly, some of these sources include industry positions, which were used to contextualize why e-LNG is being pursued. An annotated reference list is included to highlight the background and motivations for each reference.
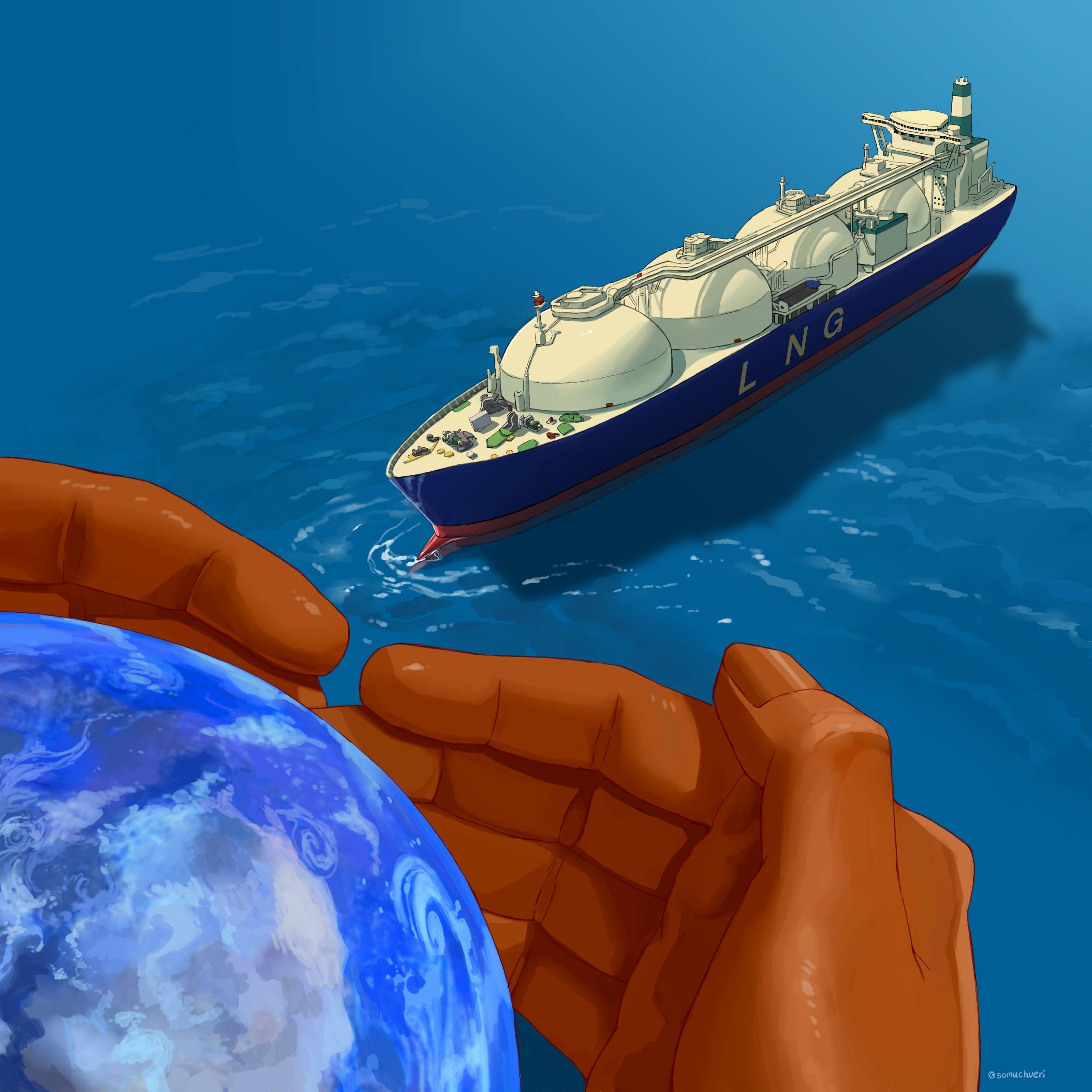
Summary
e-LNG is produced using a methanation reaction powered by ideally renewable electricity. It combines hydrogen with CO2 captured either from point CO2 (from fossil fuel plants, industry, bio-based sources, and transportation) or direct air capture.
e-LNG provides an alternative to LNG that does not rely directly on fossil fuels and utilizes the same engines, which have become increasingly more common with the global increased usage of LNG as a marine fuel.
While the use of e-LNG may lead to almost a 100% GHG reduction compared to marine gas oil, Well-to-Wake energy consumption will drastically increase.
The production of e-LNG requires large amounts of electricity, which at least for now is not sourced completely from renewables and are constrained to the type of electricity produced in the region.
The energy density required to produce e-LNG makes it less energy efficient compared to other options such as direct use of electricity.
The use of e-LNG still emits upstream methane leakage and downstream methane slip.
For e-LNG to be useful in significantly contributing to climate goals, it needs to be produced by 100% renewable energy, and the methane slip from marine engines needs to be virtually eliminated and methane leaks upstream and from LNG fuel tanks and LNG cargo tanks of ships need to be greatly reduced (ICCT 2022).
A lot of framing around industry positions seems to use idealized future scenarios of 100% renewable supply and closed loop systems to reach carbon neutrality. While the reality of today is that this is not the case, and the associated emissions to develop enough electricity to produce enough e-LNG to fuel maritime transportation would not equate to decarbonization.
* Note: The information included in the literature review to follow includes a mix of peer-reviewed and grey literature sources. Importantly, some of these sources include industry positions, which were used to contextualize why e-LNG is being pursued. An annotated reference list is included to highlight the background and motivations for each reference.
Background
The Oil and Gas industry has been strategically rebranding Liquefied Natural Gas (LNG) with a bio and e-fuel label – a greenwashed move aimed at aligning with the growing demand for green/sustainable energy sources. However, LNG variations such as bio or e-LNG are not fit to tackle climate challenges and do not align with international commitments to meet climate targets. The Say No to LNG campaign seeks to build a narrative that challenges e-LNG as a marine fuel to support its campaign and advocacy efforts. The literature review will be the start point for this work.
What is e-methane/e-LNG?
e-methane or e-LNG has been referred to as carbon-based liquid methane, synthetic renewable LNG, Synthetic Natural Gas, SNG, electro-methane, green methane, liquid green methane, liquefied synthetic methane (LSM), and hydrogen-based LNG, among other names used interchangeably within the sector and by e-LNG advocates (SeaLNG 2021). These names are all used to convey a concept termed Power-to-Gas, Power-to-Fuel, or Power-to-F (PtF). PtF converts power (electricity derived from renewable sources such as solar, wind, hydro, biomass, or geothermal)) to fuel, synthetic hydrocarbon fuels called electrofuels, or e-fuels (Nemmour et al 2023).
e-LNG is an electrofuel, meaning it is made from synthetic pathways, such as being produced from excess or additional renewable electricity and point source CO2 (ICCT 2022). It is considered to be chemically identical to biomethane (Carvalho et al. 2021), and e-LNG advocates tout it as being chemically identical to fossil LNG, while being carbon neutral (SeaLNG, 2021). While burning e-LNG produces CO2, carbon neutrality depends on a closed-loop system, where direct air capture is used to make more synthetic fuel. However, technology for direct air capture of CO2 is unlikely capable of meeting demands by 2030 (ICCT 2022).
Why is the industry interested in e-LNG?
To meet international and national GHG emission reduction targets, most notably the Paris agreement (2015), the shipping industry must reduce GHG emissions by 75-85% per ton-mile up to 2050 in order to achieve a 50% absolute reduction (Lindstad 2021). Reductions can be achieved through technical and operational improvements, as well as fuels with zero or lower GHGs (Bouman et al 2017). The most cost effective strategies to achieve this are often prioritized.
Between 2012 and 2018, global use of LNG as a marine fuel grew 28% (Faber et al., 2020), as well as the use of dual fuel internal combustion engines that allow up to 4.5% of the fuel to escape unburned in the form of methane slip (Pavlenko et al., 2020). Increased interest in LNG as a marine fuel has stemmed from efforts to comply with existing International Maritime Organization (IMO) emissions regulations (Pavlenko et al., 2020).
e-fuels are fully blendable with their fossil counterparts (e.g., e-LNG can be blended with LNG), and can be used on vessels without requiring modifications or new infrastructure (Lindstad 2021). This appears to be more cost efficient and attainable as compared to e-hydrogen or e-ammonia, which requires new vessels and supply infrastructures, or conversions of existing ones (Lindstad 2021). When positioned as carbon neutral, e-LNG appears to offer a balance between meeting GHG reduction targets with meeting customer demands and remaining economically competitive (Bouman et al. 2017), Thus, the idea of e-LNG as a carbon neutral alternative can help to justify the investment in fossil LNG-fueled ships as they can be readily switched over in the future (ICCT 2022).
How is e-methane/e-LNG made?
e-LNG is produced by combining hydrogen derived from water electrolysis with CO2, using a methanation reaction powered by renewable electricity (2H2O + renewable energy -> 2H2 + O2) (ICCT 2022). CO2 can be captured from flue gas generated by power plants, industry, and transportation, or via direct air capture. e-methane and e-LNG is distinct from bioLNG or biomethane in that it is not directly derived from biomass, although production pathways also include methane captured from biogenic sources such as landfills or manure digesters (ICCT 2022).
Hydrogen production
To increase volumetric density and make Hydrogen feasible for shipping, it must be liquified (at -253 degrees Celsius) or compressed. While compression requires less energy, the cost of building pressure tanks exceeds that of storing liquid Hydrogen (Lindstat et al. 2021). Currently, global Hydrogen production is dominated by natural gas and not renewables, a process which can increase GHG emissions with 40-66% compared to standard marine gas oil due to transformation losses when converting natural gas to Hydrogen to liquid Hydrogen (Lindstat et al. 2021). If this were to shift to producing larger amounts of global hydrogen Hydrogen through electrolysis from renewable electricity, energy consumption would increase by 10-15% on a Well-to-Wake basis, as compared to being produced from natural gas (Lindstat et al. 2021).
CO2 sources
Point sources of CO2 such as fossil fuel power plants and bioenergy plants provide a near-term source of CO2. However, relying on fossil-derived CO2 may prolong the use of fossil fuels as efforts (ICCT 2022). Direct air capture (DAC) temporarily removes CO2 from the atmosphere and re-releases it once the fuel is burned. However, the technology for DAC is still in development and is likely to be several times more expensive than point source capture, particularly in the near- term (ICCT 2022).
Energy usage
The production of e-LNG requires gas to be liquefied for use as a marine fuel, therefore using additional energy from other fuel sources (ICCT 2022). Additionally, due to thermodynamic conversion losses during the production process, a significant amount of renewable energy is required, making e-fuels, including e-LNG, less energy efficient compared to other options, such as electricity (Concawe, 2019). Fossil fuels require approximately 2.2-2.4 units of energy to deliver 1 unit on the propeller on a Well-to-Wake basis, while hydrocarbon e-fuels such as e-LNG have the highest energy usage, with 6.1-7.1 energy units of renewable electricity required to deliver 1 energy unit at the propeller. Future technology developments could reduce this by 10-15%, or 5.7-6.3 energy units. Additionally, DAC technologies are associated with high energy demands. Both heat and electricity are required for its operation, contributing to high energy needs and costs. Ultimately, switching to hydrocarbon e-fuels such as e-LNG can more than double the Well-to-Wake energy consumption compared to current fossil fuels (Lindstat et al. 2021).
GHG emissions
While e-fuels are touted as offering up to a 100% reduction in GHG emissions, this is based on assumptions that production is based on 100% renewables, which is not a reality today. Rather, e-fuels are constrained to the electricity produced in the region (Lindstat et al. 2021).
Hydrocarbon e-fuels combusted in dual fuel diesel engines may lead to almost a 100% GHG reduction compared to marine gas oil, however, Well-to-Wake energy consumption will nearly triple (140%-200% increase) (Lindstat et al. 2021).
The 2022 ICCT report found that even under a 100% ‘renewable’ LNG scenario, emissions in 2030 are 6% higher than 2019 levels if considered on a 20-year global warming potential (GWP20) basis. The report also found that absolute methane emissions would double from 2019 to 2030 due to upstream methane leakage and downstream methane slip, even when using 100% renewable LNG (ICCT 2022).
Methane slip
e-LNG has the same issues with un-combusted methane as with fossil LNG (Lindstat et al. 2021; Carvalho et al. 2021). The unburned fuel emitted from dual fuel internal combustion engines on ships, known as “methane slip”, combined with the methane leakage that happens throughout the LNG supply chain, can result in higher well-to-wake (WTW) carbon dioxide-equivalent (CO2e) emissions from ships using LNG compared with conventional marine fuels (ICCT 2022). When dual fuel engines run on e-LNG they still emit the same amount of methane slip, with upstream methane leakage varying depending on how the fuel is made (ICCT 2022). The production of e-LNG requires gas to be liquefied for use as a marine fuel, which creates more opportunities for methane leakage (ICCT 2022). While e-LNG might offer greater potential to reduce GHGs, fugitive methane emissions along the natural gas chain remain a major concern (Laskar and Giang 2023). More research is required to better understand the interplay between reduced CO2 emissions, and fugitive methane emissions (Bouman et al. 2017) as well as the rate of methane slip from marine engines when using e-LNG compared to fossil LNG.
Fuel and engine system
To combust, e-LNG requires pilot marine diesel oil, which is a distillate diesel oil that is a mixture of heavy fuel oil and gas oil, and studies have assumed that 5% pilot fuel and 95% E-fuel energy fraction is burned at the main engine (Zincir et al. 2023).
Under a high-price scenario for renewable electricity, e-LNG and other hydrocarbon e-fuels will approximately triple the total annual cost of a tanker or bulker in the 40’-80’ deadweight range compared to marine gas oil (Lindstat et al. 2021). Under a low-price scenario for renewable electricity, e-LNG may become more competitive to other e-fuels because it can be used as blend-ins in existing infrastructure and shipping fleets (Concawe, 2019).
Other challenges and limitations
Often, studies omit a full life-cycle assessment of GHG emissions when discussing e-fuels and instead apply a Well-to-Wake approach or, even more limited, Tank-to-Wake (ship only). These fail to account for the production and setup of renewable electricity generation, including the associated supply grids, end-of-production treatment, and final disposal (Lindstat 2021). This is an important consideration, as to increase the technological capacity and produce e-LNG and other e-fuels for a large-scale commercial plant, it is estimated that facilities would require scaling up by a factor of 100,000 times compared to current demonstration scales (Concawe, 2019). The associated energy consumption and emissions that this would require would need to be incorporated into any life cycle assessment of GHG emissions.
While in an idealized future, nearly all energy might come from renewables, the reality of today is that this is not the case, and lifecycle emissions remain significant, but have not been studied and quantified in full. This is an important point, given the increased demand for renewable electricity production that would be required to support demands of the transportation sector. For example, in Sweden, using e-fuels would require increasing current Swedish electricity generation by 60% to meet demands of the transportation sector (Hansson et al. 2017). Because renewable electricity is required for e-fuels to contribute to GHG reductions, a significant increase in renewable electricity production would be needed (Concawe, 2019). Thus a full life cycle analysis of scaling the production of e-fuels to meet marine shipping demands is needed in order to truly estimate GHG emissions.
When compared against other alternative maritime fuels for use in Brazil, e-LNG registered with the consistently lowest scores in a sensitivity analysis looking at availability, applicability, technological readiness, energy density, economic, safety, standards, local sustainability and global sustainability. Low scores were associated with high costs, low technological maturity, availability, and energy density (Carvalho et al., 2021).
References
While most authors do not include positionality statements or disclose any competing interests in the articles reviewed, it is important to situate the works cited within the broader context of the article aims and objectives, as well as the lead author or organizational affiliations. This extra information is included in the form of an annotated reference list.
Bouman, E.A., Lindstad, E., Rialland, A.I., Strømman, A.H., 2017. “State-of-the-Art technologies, measures, and potential for reducing GHG emissions from shipping – A
Review”. Transportation Research Part D 52 (2017), 408–421. https://doi.org/10.1016/j.trd.2017.03.022
The authors reviewed about 150 studies to provide a comprehensive overview of the CO2 emissions reduction potentials and measures published in literature. The goal of the review is to identify the most promising areas, i.e. technologies and operational practices, and quantify the combined mitigation potential. The authors conclude that there is no single measure that is sufficient to reach considerable sector-wide reductions, and that adopting and combining a large number of individual dependent and independent measures will have the most success at reducing GHG emissions by a factor of 4-6 per freight unit transported with current technologies by 2050.
The lead author is affiliated with the Industrial Ecology Programme and Department of Energy and Process Engineering, Norwegian University of Science and Technology (NTNU), which is an interdisciplinary research programme specialized in environmental sustainability analysis, including research areas focusing on climate change mitigation in the maritime sector.
Carvalho, F., Müller-Casseres, E., Poggio, M., Nogueira, T., Fonte, C., Wei, F.K., Portugal-Pereira, J., Rochedo, P.R.R., Szklo, A., Schaeffer, R. 2021. “Prospects for carbon-neutral maritime fuels production in Brazil.” Journal of Cleaner Production, October, 2021, pg. 1-11. https://doi.org/10.1016/j.jclepro.2021.129385
The authors evaluate 14 alternative fuel options for the Brazilian maritime trade to reduce GHG emissions in mid- and long-terms aligned with the IMO. They apply different weights to various technical, economic, and environmental criteria to assess alternative fuel options. They conclude that drop-in fuels such as Fischer-Tropsch diesel, alcohol-based diesel, straight and hydrotreated vegetable oils and e-diesel are mid-term alternatives, while bio-LNG is hampered by methane slip risks. Similarly, the high costs, low technological maturity, availability, and energy density associated with e-LNG make it a much less competitive alternative.
Concawe. 2019. “A look into the role of e-fuels in the transport system in Europe (2030–2050)” written by Yugo, M. & Soler, A. https://www.concawe.eu/wp-content/uploads/E-fuels-article.pdf
This literature review was written through Concawe’s Low Carbon Pathways project. It aims to build a better understanding of e-fuel production technologies and implications in terms of efficiency, greenhouse gas reduction, technology readiness level, environmental impact, investment, costs and potential demand. The authors provide an overview of advantages and disadvantages/barriers. They conclude by presenting key enablers and opportunities/synergies, implying a positive view on the potential for e-fuels.
Concawe is an organization whose membership is comprised of most oil companies in Europe. The organization aims to carry out research on environmental issues relevant to the oil industry.
Faber, J., Hanayama, S., Zhang, S., Pereda, P., Comer, B., Hauerhof, E., Schim van der Loeff, W., Smith, T., Zhang, Y., Kosaka, H., Adachi, M., Bonello, J.-M., Galbraith, C., Gong, Z., Hirata, K., Hummels, D., Kleijn, A., Lee, D., Liu, Y., … Yuan, H. 2020. Fourth IMO greenhouse gas study. International Maritime Organization. https://www.imo.org/en/OurWork/Environment/Pages/Fourth-IMO-Greenhouse-Gas-Study-2020.aspx
The report presents key findings from the Fourth IMO GHG Study, from 2020, prepared from official documents of the IMO. Written by a consortium, reviewed by the Steering Committee and expert reviewers. The contact person is Jasper Faber (CE Delft). Data was contributed by BIMCO, exactEarth, the International Energy Agency (IEA), IHS Maritime, Maersk, Stockholm Environment Institute and the World Shipping Council.
Hansson, J., Hackl, R., Taljegard, M., Brynolf, S., and Grahn, M. 2017. “The potential for electrofuels production in Sweden utilizing fossil and biogenic CO2 point sources.” Frontiers in Energy Research, March 2017, pg. 1-12. DOI: 10.3389/fenrg.2017.00004
The authors present a study that maps, categorizes, and quantifies point source CO2 emissions from industrial and combustion processes in Sweden. This is used to estimate the potential for electrofuels (power-to-gas) based on carbon capture and utilization. If all recoverable CO2 were used to produce electrofuels, it would yield approximately 2-3 times current demand for transportation fuels. However, the electricity required would amount to 3 times the electricity Sweden’s current electricity supply. Electricity required for production and associated costs are the limiting factor for potential electrofuels in Sweden. At scale, this requires a substantial amount of renewable electricity at a relatively low cost.
The lead author is affiliated with Climate and Sustainable Cities, IVL Swedish Environmental Research Institute, Stockholm, Sweden, which conducts grant based and consultancy based research on environmental and sustainability issues. The author is also affiliated with the Division of Physical Resource Theory, Department of Energy and Environment, Chalmers University of Technology, Göteborg, Sweden, which researches sustainable energy systems and society.
ICCT (International Council on Clean Transportation). 2022. Comparing the future demand for, supply of, and life-cycle emissions from bio, synthetic, and fossil LNG marine fuels in the European Union. Report prepared by Comer B, O’Malley J, Osipova L, and Pavlenko N. https://theicct.org/wp-content/uploads/2022/09/Renewable-LNG-Europe_report_FINAL.pdf
The report estimates demand for LNG used by ships on voyages to, from, and between EU ports in 2030. The predicted demand is then compared to estimates of the potential supply of renewable LNG and the life-cycle WTW GHG emissions calculated based on various proportions of renewable LNG. The authors are the International Council on Clean Transportation, an independent nonprofit research organization. This report was funded by the ClimateWorks Foundation.
Laskar, I.I., and Giang, A. 2023. “Policy approaches to mitigate in-use methane emissions from natural gas use as a marine fuel.” Environmental Research Infrastructure and Sustainability, May 2023, pg. 1-20. https://doi.org/10.1088/2634-4505/accf33
The authors identify methane slip mitigation measures, and then identify and evaluate potential policy instruments that could incentivize their uptake while considering the shipping sector’s climate targets. They find that regulatory instruments will likely perform best at the global level, including methane on a CO2-equivalent and lifecycle basis.
The lead author is affiliated with the Institute for Resources, Environment, and Sustainability at the University of British Columbia.
Lindstad, E., Lagemann, B., Rialland, A., Gamlem, G.M., Valland, A. 2021. “Reduction of maritime GHG emissions and the potential role of E-fuels.” Transportation Research Part D, November 2021, pg. 1-15. https://doi.org/10.1016/j.trd.2021.103075
The authors provide an analysis of E-fuels including emissions (GHG reduction potential), cost, and energy use. The goal of the paper is to perform a transparent Well-to-Wake assessment of e-fuels and associated engine technologies, including fuel tank systems, and to document that a maritime-focused perspective on emissions and zero carbon fuels oversees impacts on global energy supply.
The lead author is affiliated with SINTEF Ocean AS, an organization that conducts research related to ocean space for national and international industries, including maritime transportation. The organization states that “Zero-emission fuels are an important part of the solution, but the supply and price of these fuels will be a challenge. Therefore, it will become even more important in the future to reduce the energy consumption of ships through better design of hulls and propellers, efficient energy systems and optimisation of logistics and operations. These are areas that SINTEF Ocean focuses on, and where we connect technology expertise with expertise in digitalisation and autonomy solutions.”
Nemmour, A., Inayat A., Janajreh, I., Ghenai C. 2023. “Green hydrogen-based E-fuels (E-methane, E-methanol, E-ammonia) to support clean energy transition: A literature review.” International Journal of Hydrogen Energy, April 2023, pg. 29011-29033. https://doi.org/10.1016/j.ijhydene.2023.03.240
The authors conducted a literature review on e-fuels in the context of supporting a clean energy transition. They provide an overview of the development of PtF technologies, including research on e-fuel production from renewable sources, and research focusing on carbon capture, water electrolysis and conversion routes for PtF. They highlight a number of barriers and limitations to successful implementation. Limitations include 1) the high cost of renewable resources related to building and installing facilities, availability of power, infrastructure, safety, transportation, and supply; 2) the high cost of green hydrogen production (2-3 times that of ‘blue’ hydrogen (fossil fuels + CCS) and grey hydrogen (steam reforming of natural gas); 3) large scale e-fuels necessitate increased demand for electricity production; 4) intermittent and fluctuating power output from renewable energy sources requires a vigorous electrical grid to be integrated to allow for electro-fuel plants to operate regularly; 5) energy and carbon losses losses during storage and transport of green hydrogen and electro-fuels; 6) sustainability of water availability, given the vast amounts of water required to produce hydrogen using electrolysis. Despite these limitations, the authors conclude that the production of e-fuels remains promising, with the key to decarbonizing the global economy as tackling the barriers and limitations they presented.
The lead author is affiliated with the Biomass and Bioenergy Research Group, Sustainable Energy and Power System Research Centre, Research Institute for Sciences and Engineering, University of Sharjah, Sharjah, United Arab Emirates. The group aims to “aims to research advances in technology to address potential green industries’ demands”.
Nicitia, A., Maggio, G., Andaloro, A.P.F., Squadrito, G. 2020. “Green hydrogen as feedstock: Financial analysis of a photovoltaic-powered electrolysis plant.” International Journal of Hydrogen Energy, April 2020. Pg. 11395-11408. https://doi.org/10.1016/j.ijhydene.2020.02.062
The authors present a proposed case-study for a hypothetical 200kW photovoltaic plant and 180 kW electrolyser, and do a techno-economic-financial evaluation of this hypothetical system to produce green hydrogen to be sold as a feedstock for industries and research centers. The analysis is focused on small- and medium-sized local market demands for technical gasses, and not production potential related to the marine transportation industry. Based on their analysis, the authors conclude that return on investment and profitability are achievable (over 12 years) if oxygen is sold in addition to hydrogen, and that there are reasons to believe that hydrogen distributed production could have a broad deployment and use in the future.
The lead authors is affiliated with L’Istituto di Tecnologie Avanzate per l’Energia “Nicola Giordano” (ITAE), which was founded by the Italian National Research Council in 1980 with the name of Research Institute on Chemical Methods and Processes for the Transformation and Accumulation of Energy. One of the thematic research areas of the institute is electrochemical conversion of renewable energy into hydrogen and alternative fuels.
Pavlenko, N., Comer, B., Zhou, Y., Clark, N., & Rutherford, D. 2020. The climate implications of
using LNG as a marine fuel. International Council on Clean Transportation. https://theicct.org/
publication/the-climate-implications-of-using-lng-as-a-marine-fuel/
This working paper compares the life-cycle GHG emissions of LNG, marine gas oil (MGO), very low sulfur fuel oil, and heavy fuel oil when used in engines suitable for international shipping, including cruise ships. The authors are the International Council on Clean Transportation, an independent nonprofit research organization. This paper was funded by Stand.earth.
Sea-LNG. 2021. Let’s Call It Renewable Synthetic LNG. Accessed June 14, 2024. https://sea-lng.org/2021/12/lets-call-it-renewable-synthetic-lng/
The post provides a brief overview of e-LNG and addresses the different terms that have been used to describe it, advocating for the use of “Renewable Synthetic LNG”.
Sea-LNG is an organization that advocates LNG as a solution to all of the challenges that the shipping industry faces through tighter environmental regulations.
Zincir, B.A., Zincir, B., Usluer, H.B., Arslanoglu, Y. 2023. “Using an E-fuel Method to Meet the 2030 Decarbonization Target: A Case Study.” In: Sogut, M.Z., Karakoc, T.H., Secgin, O., Dalkiran, A. (eds) Proceedings of the 2022 International Symposium on Energy Management and Sustainability . ISEMAS 2022. Springer Proceedings in Energy. Springer, Cham. https://doi-org.ezproxy.library.dal.ca/10.1007/978-3-031-30171-1_51
The authors present a case study comparing fossil fuel, fossil-based alternative fuels, and E-fuels using a general cargo ship. The authors note that from the perspective of CO2e emission, E-diesel is the leading E-fuel, and from the fuel consumption perspective, E-LNG is the prominent E-fuel with lower voyage-based fuel consumption. They conclude that E-diesel is the best solution, although fuel costs are much higher than marine diesel oil.
The lead author is affiliated with the Maritime Faculty, Istanbul Technical University, Istanbul, Tuzla, Turkey.
References for Industry Positions:
Tokyo Gas – Advertisement Feature published in Nature
https://www.nature.com/articles/d42473-022-00166-2
Sea-LNG
https://sea-lng.org/2021/12/lets-call-it-renewable-synthetic-lng/
World Economic Forum, post written by Marco Alverà CEO and Co-Founder of Tree Energy Solutions (e-LNG producer)
https://www.weforum.org/agenda/2024/01/eng-synthetic-natural-gas-decarbonize-shipping/
For media Inquiries please contact [email protected].